Exploring synthetic engineering of bacteriophages
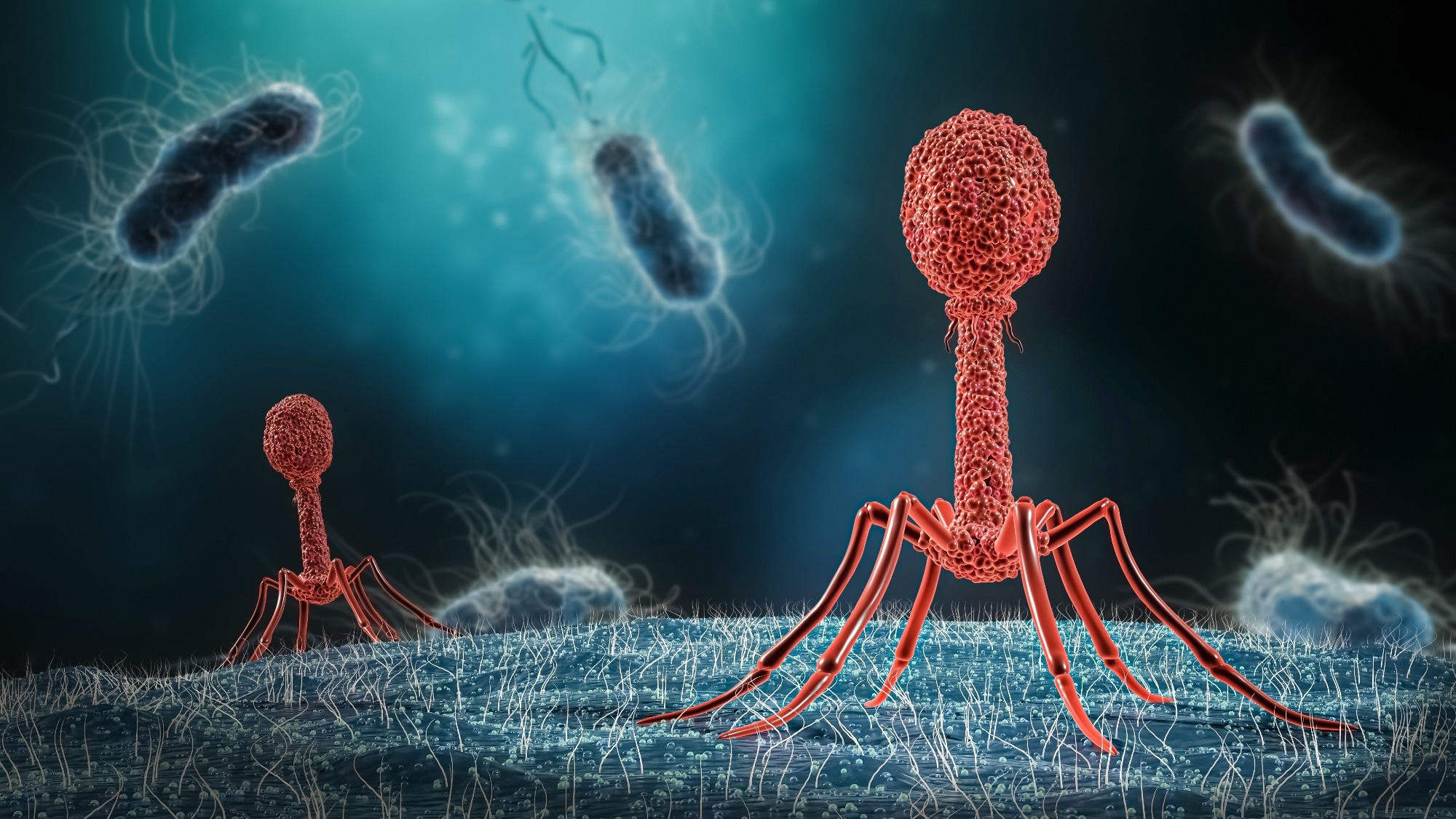
In a current research posted in the Proceedings of the Countrywide Academy of Sciences, researchers demonstrated biological bacteriophage containment and artificial engineering.
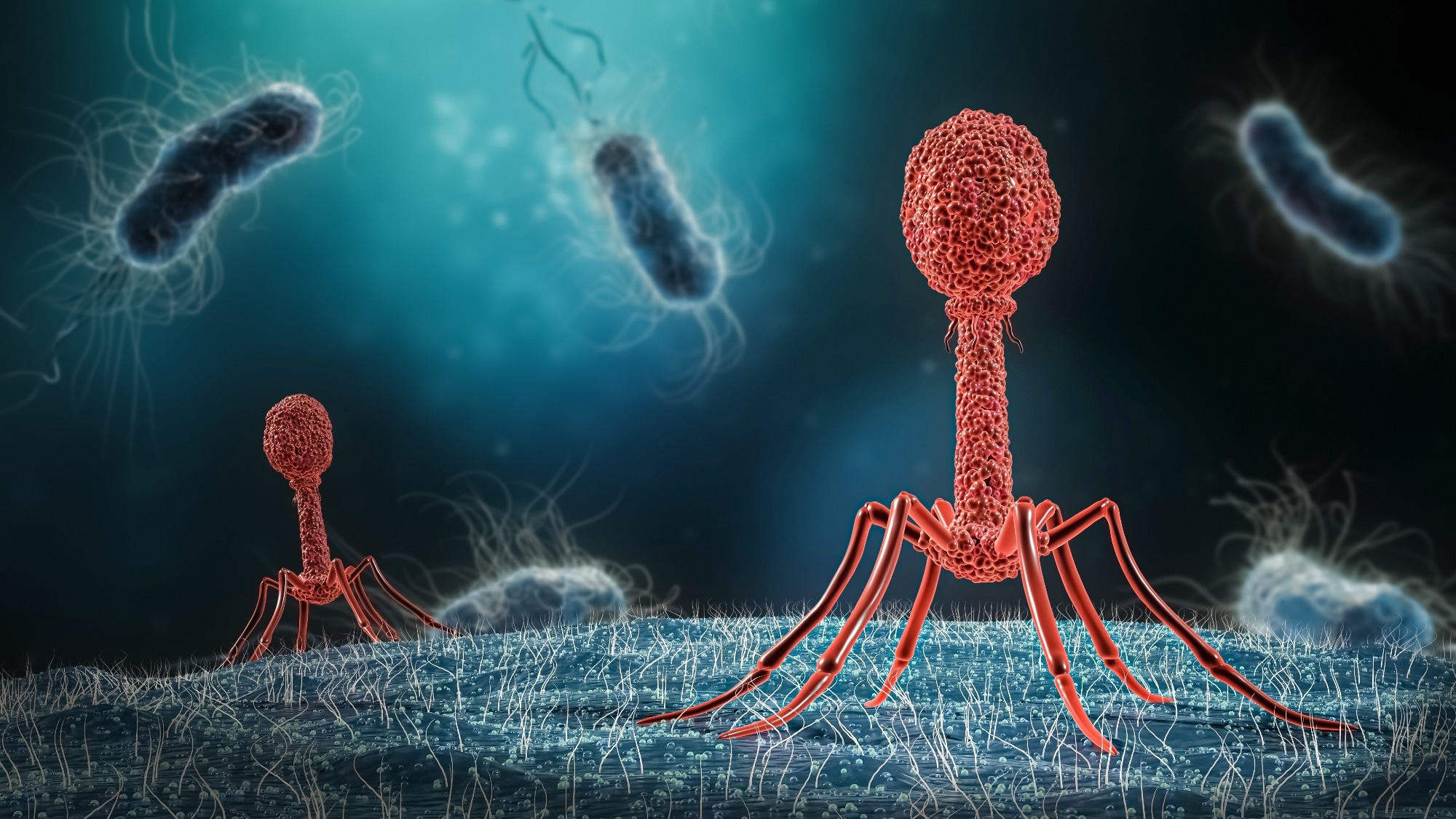
The grave hazards posed by drug-resistant bacterial health conditions and current advancements in artificial biology have pushed substantial attention to genetically modified phages with therapeutic opportunity. So much, numerous studies on modified phages have been confined to evidence-of-idea (POC) or foundational research employing phages with relatively brief genomes or “phage exhibit kits.” In addition, no safeguards ensuring productive translation for simple software have been carried out.
About the review
The current review created a platform for mobile-no cost phage engineering and rebooting.
Very first, the staff sought to reboot numerous wild-sort (WT) phages that infected gram-unfavorable germs and acid-rapidly mycobacteria utilizing genomes assembled in vitro. Polymerase chain reaction (PCR) was used to amplify the completely assembled phage genome obtained from either chemically-generated deoxyribonucleic acid (DNA) or template phage genome. Primers ended up designed to crank out 28 bp to 65 bp homologous places in each and every adjacent PCR fragment. These items have been assembled and electroporated into bacterium hosts, ensuing in the reactivation of phages.
The team designed fragments that enabled close-joining to reboot phages derived from round genomes. Coliphage lambda was properly reactivated from five fragments in Salmonella phage P22 and E. coli, along with four fragments existing in S. Typhimurium LT2. The product mycophage D29, which infects acid-rapid Mycobacterium spp and has a higher guanine-cytosine genome, was also rebooted. 5 DNA fragments were manufactured to make the circular genome.
Effects
The product coliphage T7, acquiring a linear genome, exhibited linear concatemerization at the replication time and was employed to examine the study system. The linear genome was created from 4 PCR fragments and then electroporated into E. coli to reactivate the phage T7. Coliphage T3, Pseudomonas phage gh-1, and Salmonella phage SP6 ended up predicted to have genomic architectures and lifestyle cycles equivalent to individuals of T7. The group also efficiently regenerated T3 from E. coli DNA fragments, SP6 from Salmonella Typhimurium LT2 DNA fragments, and gh-1 from Pseudomonas putida DNA fragments. These outcomes demonstrated that the study style guideline and methodology were powerful for rebooting phages from assembled linear genomes.
The purposeful mycophage D29, an uncharacterized mycophage B1, and GS4E have been rebooted from M. smegmatis mc2 155. Mycophage TM4 was produced from 6 chemically generated DNA segments based on its publicly accessible sequence information. The DNA fragments underwent amplification to demonstrate a 28 bp to 38 bp overlap with adjacent parts, purified, and then circularized in vitro. The genome was released into M. smegmatis mc2 155. The working artificial TM4, whose genome was chemically produced, was rebooted. The full artificial TM4 genome was sequenced by MiSeq and verified to be 100{64d42ef84185fe650eef13e078a399812999bbd8b8ee84343ab535e62a252847} equivalent to the referred TM4, indicating helpful phage rebooting from sequence knowledge.
The properly-characterised phage T7 was chosen as a model for the POC investigation. The T7 machinery identified a packaging sign sequence (Pac) to “package” or “retail outlet” its genome in a T7 head particle. Pac comprises PacB and PacC. Terminase modest subunit gp18 binds with the subunit gp19–prohead elaborate for DNA translocation into phage head. Pursuing headful packaging, the staff famous that gp19 cleaves at PacC in the concatemeric T7 phage genome, main to T7 head maturation.
Then, a plasmid made up of the Pac and “your favourite gene” (yfg) was created and injected into E. coli. Electroporation of the genome into the E. coli cells containing the plasmid created synthetic transducing particles centered on T7 (T7Pac). In the mobile, the genome devoid of Pac generated progeny virions right before packaging the plasmid containing Pac into head particles, yielding T7Pac-yfg. Working with this method, the workforce synthesized T7Pac-lacZ, a plasmid harboring lacZ. The lysate was mixed with lacZ-deficient E. coli, adopted by plating with LB X-gal plates. Consequently, 1.6×104 CFUs/mL of T7Pac-lacZ were being produced, and all colonies that shaped were being blue. On the bacterial garden, T7Pac-lacZ did not develop plaques, suggesting organic containment.
The crew developed the SP6 phage genome minus the capsid protein-encoding gene 31. The genome was launched into the LT2 pressure of S. Typhimurium, which expressed gene 31. Employing PCR, the deletion of gene 31 from the synthetic SP6Δhead was verified. The received higher titer SP6Δhead lysate formulated plaques on a lawn of LT2 that expressed gene 31, which had been not present on the parental LT2.
General, the study conclusions demonstrated the generation of normal and engineered phages that infect gram-negative germs and acid-rapid mycobacteria by means of in vitro genome assembly.