From mechanobiology to mechanical repair strategies in IVD
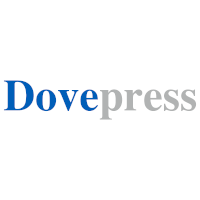
Dian Zhang,1,2,* Minshan Feng,1,* Wei Liu,3,* Jie Yu,1 Xu Wei,1 Kexin Yang,1 Jiawen Zhan,1 Wei Peng,1,2 Mingyi Luo,1,2 Tao Han,1 Zhefeng Jin,1 He Yin,1 Kai Sun,1 Xunlu Yin,1 Liguo Zhu1
1Department of Spinal Surgery, Wangjing Hospital of China Academy of Chinese Medical Sciences, Beijing, People’s Republic of China; 2Wangjing Hospital of China Academy of Chinese Medical Sciences, Beijing University of Chinese Medicine, Beijing, People’s Republic of China; 3Department of Nursing, Shandong University of Traditional Chinese Medicine, Jinan, People’s Republic of China
Correspondence: Xunlu Yin; Liguo Zhu, Department of Spine, Wangjing Hospital of China Academy of Chinese Medical Sciences, Beijing, 100102, People’s Republic of China, Email [email protected]; [email protected]
Abstract: Neck pain and low back pain are major challenges in public health, and intervertebral disc (IVD) biomechanics is an important multidisciplinary field. To date, no bibliometric literature review of the relevant literature has been performed, so we explored the emerging trends, landmark studies, and major contributors to IVD biomechanics research. We searched the Web of Science core collection (1900– 2022) using keywords mainly composed of “biomechanics” and “intervertebral disc” to conduct a bibliometric analysis of original papers and their references, focusing on citations, authors, journals, and countries/regions. A co-citation analysis and clustering of the references were also completed. A total of 3189 records met the inclusion criteria. In the co-citation network, cluster #0, labeled as “annulus fibrosus tissue engineering”, and cluster #1, labeled as “micromechanical environment”, were the biggest clusters. References by MacLean et al and Holzapfel et al were positioned exactly between them and had high betweenness centrality. There existed a research topic evolution between mechanobiology and mechanical repair strategies of IVDs, and the latter had been identified as an emerging trend in IVD biomechanics. Numerous landmark studies had contributed to several fields, including mechanical testing of normal and pathological IVDs, mechanical evaluation of new repair strategies and development of finite element model. Adams MA was the author most cited by IVD biomechanics papers. Spine, the European Spine Journal, and the Journal of Biomechanics were the three journals where the most original articles and their references have been published. The United States has contributed most to the literature (n = 1277 papers); however, the research output of China is increasing. In conclusion, the present study suggests that IVD repair is an emerging trend in IVD biomechanics.
Introduction
Neck pain and low back pain are major challenges in public health, with prevalence rates of nearly 3.6{64d42ef84185fe650eef13e078a399812999bbd8b8ee84343ab535e62a252847} and 7.0{64d42ef84185fe650eef13e078a399812999bbd8b8ee84343ab535e62a252847} and affecting 288.7 million and 570 million people worldwide, respectively.1,2 Specifically, low back pain is the primary contributor to years lived with disability among all diseases.2 Diseases of the intervertebral discs (IVDs), such as degeneration, are important causes of pain, and >400 million people are diagnosed with symptomatic disc degeneration worldwide each year.3
Normal IVDs are kidney-bean shaped structure lying between adjacent vertebral bodies. The height and diameter of them are approximately 7–10 mm and 4 cm respectively.4 In each IVD, there is a jelly-like core, the nucleus pulposus (NP), surrounded by a tire-like structure called the annulus fibrosus (AF), both of which are sandwiched by the cartilage end plates (CEPs).5 Mechanical function is the major component of IVD physiology. Due to well-designed architecture and interaction of their three main components, IVDs can provide support and flexibility to the spine, allowing it to bend, twist, distribute compression and absorb shock.
The tissues of IVDs are mainly composed of water, proteoglycans, and collagen. The relative content of them is different between NP, AF and CEPs, resulting in distinctive mechanical properties. The NP has the highest proteoglycan content which accounts for roughly 50{64d42ef84185fe650eef13e078a399812999bbd8b8ee84343ab535e62a252847} of the dry weight, leading to a high water content (75–90{64d42ef84185fe650eef13e078a399812999bbd8b8ee84343ab535e62a252847}) and a predominantly hydrostatic behavior.6 The negatively charged proteoglycans provides an osmotic potential,7 which is then converted into hydrostatic pressure through hydration. Intradiscal pressure provides the tissue with high compressive properties by supporting the CEPs and tensioning the AF. Normal human NP has an effective aggregate modulus of 1.0 MPa in confined compression.8 Intradiscal pressure presents a diurnal change according to the fluctuating loading of IVDs. Resting intradiscal pressure is roughly 0.1–0.24 MPa at night and increases to 0.3–1.1 MPa when standing or sitting, and to 2.3 MPa when lifting a 20-kg weight in a flexed position.9,10
AF is a complex structure made up of 15–25 highly organized concentric layers,11 which are composed of alternatingly aligned oblique collagen fibers oriented at ±25–45° to the horizontal plane and interspersed with proteoglycans.5 Due to its high collagen content which is approximately 50–70{64d42ef84185fe650eef13e078a399812999bbd8b8ee84343ab535e62a252847} of the dry weight,12 the AF has a superior tension-bearing capacity. According to the direction of loading, the average tensile modulus of AF is approximately 0.2–183 MPa.5 The concentric layers of AF are interconnected through a network of smaller fibers, which provides superior capacity to resist the shear by intra-lamellar skewing.13 Multi-scale architecture also provides prominent mechanical anisotropy and nonlinearity to AF. From the outer to the inner annulus, there is a decrease in the ratio of collagens I to II and in collagen fiber angle. The spatial variations in structure and composition lead to its anisotropy.14 Crimp of the collagen fibers in AF provides the nonlinearity.15 When the fibers are stretched, the fibers progressively straighten with minimal resistance and the tensile stress–strain curve is nonlinear. After the fiber has been straight, it starts taking load and the stress–strain curve become linear. Nonlinearity is important to permit both disc motion and stability.
Biomechanics also play an important role in the pathogenesis and therapeutics of many disc disorders. In terms of pathology, altered biomechanics can directly affect IVD cell anabolism and catabolism. Furthermore, a degraded extracellular matrix can influence the biomechanical behavior of IVDs, such as by reducing the intradiscal pressure and the ability to retain water under compressive forces.16 As a result, the mechanical characterization of IVDs can help to elucidate mechanisms of IVD disorders and understand how the material properties of IVDs are influenced by these disorders. Using this knowledge, novel biomaterials can then be designed and clinically implemented.
IVD biomechanics is a rapidly developing and important interdisciplinary discipline of spinal surgery. While many biomechanical and mechanobiological behaviors of native IVDs and tissue engineering scaffolds have been recorded by in vitro and in vivo studies, IVD biomechanics is still an active domain. However, a comprehensive understanding of IVD biomechanics remains an important gap in the literature. The present study aims to explore the emerging trends, landmark studies, and major contributors of IVD biomechanics over several decades using bibliometric analysis, a widely used quantitative method to understand the knowledge structure of a specific domain.17–19 In particular, citation count is widely used as a metric by bibliometric studies to determine the impact of a portion of peer-reviewed papers.20 However, the overall citation count of a study is not indicative of its impact on a specific domain. Many citations of papers in IVD biomechanics have been contributed by papers in other subfields of orthopedics or clinical neurology, and highly cited papers of IVD biomechanics may not have actually had the level of impact on the field that their citation count suggests. Therefore, we also pay close attention to citations contributed by IVD biomechanical studies to find out the foundation supporting this field, which is an important feature of the present study.
Materials and Methods
Data Collection
The present study performs a bibliometric analysis and review of IVD biomechanical literatures included in Web of Science core collection, which is one of the largest and most reputable global citation databases, as well as a widely used database for bibliometric analysis.17–19 The search strategy and flowchart of literature selection are shown in Table 1 and Figure 1, respectively. This study only included records of which documents are original research articles, review articles, early access papers, or proceedings papers. As a result, book chapters, meeting abstracts, editorial materials, letters corrections, and books were eliminated from consideration. Furthermore, papers not published in the English language were excluded. The process of searching and exporting papers was completed by two researchers independently to reduce errors.
![]() |
Table 1 Search Strategy Used to Identify Original Papers of IVD Biomechanics in the Web of Science Core Collection |
![]() |
Figure 1 Flowchart of literature selection in this study. |
Data Analysis
All records included were imported into CiteSpace, which is a popular bibliometric software program designed by Chen et al using the Java language,21 to perform a citation analysis.
The major contributors of IVD biomechanics were determined by research output and citation counts of authors, journals, and countries. Research outputs were available in the Web of Science. Citation counts of contributors in corresponding time slices were computed by CiteSpace.
Specifically, we established a co-citation network and performed clustering of the references. Based on the co-citation analysis, the landmark work, evolution of research topics, and emerging trends in IVD biomechanics were identified. The CiteSpace parameters of the co-citation network used by this study were as follows: timespan, January 2000–December 2022; time slice, 1 year; references selected criteria in each time slice, the top 50 most-cited references; and algorithm of the link strength between nodes, cosine.
In a bibliometric analysis, if two references (cited articles) are cited by a third article (citing article), then these two references have a co-citation relationship.22 Based on this principle, we formed a co-citation network of references of IVD biomechanics. In the network, nodes represented references and a connection between them represented a co-citation relationship. Furthermore, a clustering analysis was performed in which the co-citation network was decomposed into many heterogeneous clusters. References within the same cluster were considered tightly connected, while those between different clusters were considered to be less connected.23 Labels of co-citation clusters were extracted from the titles of citing articles by the log-likelihood ratio (LLR) test algorithm.24 By observing the time range of co-citation clusters and corresponding labels, the evolution of research topics in IVD biomechanics over time could be mapped.
Emerging trends of IVD biomechanics were determined by detecting citation bursts, which indicated that some references were associated with a sharp increase in citations. Evidently, these references have attracted more attention from other researchers than those without citation bursts. If a cluster contains a lot of nodes with citation bursts, then the cluster is more likely to capture an emerging trend.
Results
Papers of IVD Biomechanics
Overview of Publications
A total of 3189 publications were included. A paper published in 1946 ultimately did not correlate with the topic of this study; thus, the oldest paper in IVD biomechanics included herein was published by Brown et al in 1957.25 From 1957 to 2022, the number of papers in biomechanics of IVDs showed an overall upward trend (Figure 2), and the upward speed increased significantly after 2002. In 2018, the number of publications reached its peak.
Papers with the Most Annual Average Citation Counts in the Field of IVD Biomechanics
There were 119 original IVD biomechanics papers that had each been cited ≥119 times. The most cited paper was “New in vivo measurements of pressures in the intervertebral disc in daily life”, which was published in 1999 by Wilke et al9 (Table 2) and tested human intradiscal pressure under different positions. Its data were then used by Vergroesen et al16 to discuss the effect of the osmotic potential generated by proteoglycans translating into biomechanical hydrostatic pressure in healthy discs, finally leading to the conclusion that the increased fragmentation of aggrecan and reduced effective negative charge in degenerating discs may decrease the intradiscal pressure and lead to a reduction in disc height.
![]() |
Table 2 Top 10 Original Papers in IVD Biomechanics with the Highest Annual Average Citation Counts |
References Cited by IVD Biomechanical Studies
The Co-Citation Network of References and its Clustering
The modularity of the co-citation network was 0.8204, which means that references within the same cluster were co-cited much more frequently than references between clusters.23 Every cluster in Table 3 was highly homogeneous, with the lowest silhouette score being 0.825.
![]() |
Table 3 Largest Clusters in the Co-Citation Network |
The largest cluster (#0) had 148 members and we labeled it as “annulus fibrosus tissue engineering” by LLR (Figure 3 and Table 3), while the second-largest cluster (#1) which contained 144 members was labeled as “micromechanical environment.” The most relevant citer to cluster #0 was published by Iatridis et al,26 who cited 32 (21.6{64d42ef84185fe650eef13e078a399812999bbd8b8ee84343ab535e62a252847}) references of cluster #0. The most relevant citer to cluster #1 was published by Setton et al,27 who cited 27 (18.8{64d42ef84185fe650eef13e078a399812999bbd8b8ee84343ab535e62a252847}) references of cluster #1.
![]() |
Figure 3 Co-citation network of the references and its clustering. Nodes in the network represent references, and their size indicates citation counts contributed by IVD biomechanical studies. A node may have a number of rings with different colors, which means that they were cited in different time slices.23 Connections represent co-citation relationships. |
We found that clusters #2, #5, #6, and #7 were the most recent clusters, which were labeled as “ovine lumbar”, “digital volume correlation”, “herniation risk”, and “total disc arthroplasties”, respectively (Table 3). The suboptimal labels of these clusters by LLR were “complex loading condition” (LLR = 442.64, P = 0.0001), “intervertebral disc annulus” (LLR = 483.68, P = 0.0001), “annulus fibrosus repair” (LLR = 601.33, P = 0.0001), and “two-level cervical disc replacement” (LLR = 340.03, P = 0.0001), respectively.
The References with the Highest Citation Counts Contributed by Papers of IVD Biomechanics
The top 10 references highly cited by papers of IVD biomechanics from 2000 to 2022 are shown in Table 4. Half of them existed in cluster #0 (“annulus fibrosus tissue engineering”). The most cited reference was “Biomechanics of the human intervertebral disc: a review of testing techniques and results” by Newell et al,5 followed by the papers of Dreischarf et al28 and Rohlmann et al.29
Citation Bursts
Papers with strong citation bursts mainly existed in clusters #0–2 and #6 (Figure 4). The top 10 of these papers are shown in Table 5. We found that Newell et al5 and Dreischarf et al28 had the strongest citation bursts. Except for Galbusera et al30 and O’Connell,31 another eight references also existed on the list of highest citations.
![]() |
Table 4 References Most Highly Cited by Papers of IVD Biomechanics |
![]() |
Table 5 Top 10 References with the Strongest Citation Bursts |
![]() |
Figure 4 Citation bursts in the co-citation network. Red rings around the nodes represent the years when citation bursts can be found. |
Betweenness Centrality
Table 6 shows references that have the highest betweenness centrality scores. The study by MacLean et al in cluster #1 was the top-ranked reference, which involved a mechanobiological investigation and reported that cells of the NP and AF have heterogeneous mechanobiological behavior in vivo.32 This study’s corresponding node in the co-citation network was positioned between clusters #0 and #1 (Figure 5). Notably, this kind of position may become a bridge of research topic evolution.23 The paper by Holzapfel et al33 in cluster #0 had a similar position as that of MacLean et al32 between clusters #0 and #1 (Figure 5); Holzapfel et al tested the mechanical behavior of lamellae of human annulus fibrosus in vitro.
![]() |
Table 6 References with the Highest Betweenness Centrality in the Co-Citation Network |
![]() |
Figure 5 Nodes positioned between clusters #0 and #1. There were five nodes with highest betweenness centrality highlighted by purple rings, where the thickness indicated the strength of betweenness centrality. Specifically, MacLean et al (2005)32 and Holzapfel et al (2005)33 were positioned between clusters #1 and 0, which are more likely to provide insights into topics evolution or emerging trends, while the other highlighted nodes were highly connected to other nodes within the same cluster, which may make them less important than the former. (A) Spotlight model of the co-citation network, which highlights nodes with high betweenness centrality. Close-ups of MacLean et al (2005)32 (B) and Holzapfel et al (2005)33 (C), which are positioned between clusters #0 and #1. |
Other nodes of high betweenness centrality (Table 6) were highly connected to other nodes in an intra-cluster fashion, rather than between clusters. As a result, they may be considered very important in their clusters; however, they cannot help as much with the transition between research topics.
Major Contributors
Authors of Original Papers and Their References
Among the original papers included in this investigation, there were 90, 81, and 80 papers on whom Wilke HJ, Elliott DM, and Iatridis JC were authors, respectively. Meanwhile, Adams MA, Panjabi MM, and Wilke HJ were the authors most commonly found among the references of IVD biomechanical studies (Table 7), having been cited 917, 747, and 692 times up to January 2022, respectively. From January 2002–December 2021, Adams MA, Panjabi MM, Wilke HJ, Iatridis JC, Goel VK, and Urban JPG were among the 10 authors most cited by papers of IVD biomechanics in each 5-year slice. Of these, Adams MA from the University of Bristol was cited the most.
![]() |
Table 7 Top 10 Authors with the Highest Citation Counts Contributed by Papers of IVD Biomechanics |
Major Journals
As shown in Table 8, Spine was the most productive journal, having published 414 papers of IVD biomechanics, followed by the Journal of Biomechanics (J Biomech) (208 papers) and the European Spine Journal (Eur Spine J) (158 papers). Spine, Eur Spine J, and J Biomech were also determined to be the most cited journals, having been cited 2689, 2018, and 1960 times by papers of IVD biomechanics, respectively, from 2000 to 2022. From January 2002–December 2021, they were also the three most cited journals in each 5-year slice (Figure 6).
![]() |
Table 8 Top 10 Most Productive Journals in IVD Biomechanics |
Distribution of Countries/Regions in Papers of IVD Biomechanics
Up to January 2022, authors from the United States had published 1278 papers of IVD biomechanics, followed by authors from the People’s Republic of China and Canada, who published 456 and 260 papers, respectively. Similarly, the United States and the People’s Republic of China were ranked first and second in terms of scientific output from 2012 to 2016 and 2017 to 2021 (Table 9).
![]() |
Table 9 Most Productive Countries/Regions in Two Recent 5-Year Time Slices |
Discussion
For the present study, a primary purpose is to explore the evolution of research topics, and emerging trends based on the co-citation network and its clustering. Cluster #0 and #1, the largest two clusters, were labeled as “annulus fibrosus tissue engineering” and “micromechanical environment” respectively (Table 3). These labels were extracted from the titles of citing articles by the LLR algorithm.24 Specifically, the citing articles of cluster #1 were mainly concerned with investigating the micromechanical effects on the IVD cells. Among them, Setton et al27 had cited 27 (18.8{64d42ef84185fe650eef13e078a399812999bbd8b8ee84343ab535e62a252847}) references of cluster #1, being the most relevant citer. Setton et al reviewed the knowledge of micromechanical factors in the IVDs and their role in cell biology, with a key point on the differences between AF and NP. Citers of cluster #0 had a strong focus on tissue engineering. The most relevant citing article was written by Iatridis et al26 which had cited 32 (21.6{64d42ef84185fe650eef13e078a399812999bbd8b8ee84343ab535e62a252847}) references of cluster #0. Iatridis et al reviewed the key targets to repair and the promising biomaterials. Considering the statistical results and actual content of citers, the labels of cluster #0 and #1 are both acceptable.
A major evolution of research topics in the co-citation network was found between cluster #1 and #0. First, these were the largest two clusters in this study, which indicate that research supported by these references are major topics of IVD biomechanics. Second, the average publication years of references in these two clusters were 2000 and 2007, respectively, which are distinct and provide a probability of transition. Most importantly, we found nodes of high betweenness centrality (MacLean et al32 and Holzapfel et al33) between them by which they are connected tightly (Figure 5). This evolution may represent the process of the key research topic of IVD biomechanics changing from mechanobiology to tissue engineering. This result is logical as progress in mechanobiology can help to guide the strategy and design of functional engineered tissues.34 In this study, cluster #0 was comprised of highly concentrated nodes with citation bursts (Figure 4). The citation years of references in cluster #0 ranged from 2007 to 2015, indicating that cluster #0 attracted much attention and captured the emerging trend of IVD biomechanical research at that time.
Clusters #5 and #6 were the two most recent clusters in this study (Table 3 and Figure 3), and cluster #6 was also comprised of highly concentrated nodes with citation bursts (Figure 4). It is evident that references in cluster #6 have attracted extensive attention at present, and cluster #6 probably captured the current emerging trend. The optimal label of cluster #6 was “herniation risk”, and the suboptimal label was “annulus fibrosus repair.” The most relevant citer published by Fujii et al35 had cited 15 (15.5{64d42ef84185fe650eef13e078a399812999bbd8b8ee84343ab535e62a252847}) references of cluster #6. They tested the biomechanical properties of AF repaired by genipin crosslinked fibrin adhesive hydrogel. The second relevant citer provided a systematic review of injectable cell delivery biomaterials used in IVD repair.36 Therefore, the actual interests of the citing articles were consistent with the suboptimal label of clusters #6, “annulus fibrosus repair”.
As presented in cluster #0 and #6, AF tissue engineering and repair had been identified as emerging trends of IVD biomechanics. However, their citing articles investigated the NP replacement as well. For example, the most relevant citing article as well as a highly cited article of cluster #6, had reviewed the promising materials both for AF repair and NP replacement.26 Most importantly, there is an objective expansion in the studies about NP replacement. Therefore, considering “IVD repair” as scope of the emerging trends was conservative, but more reasonable for the present study. Moreover, the precise scope of “repair” should be determined. Biological repair strategies for intervertebral disc degeneration can be classified into three categories: biomolecular therapy, cell therapy, and biomaterial-based therapy.37,38 According to the labels and primary citing articles26,35,36 of cluster #0 and #6, which was discussed before, the identified emerging trend was more relevant to biomaterial field rather than biomolecular/cell field. This finding is in line with the mainstream38 and will be discussed below.
Biomechanics serves to evaluate new repair strategies, for example, comparing the mechanical properties between new tissue engineering scaffolds and native tissues. Therefore, it is crucial to establish a goal for repair strategies, based on mechanical properties of native IVDs. Considering this, a review article by Nerurkar et al39 (in cluster #0, with 32 citations contributed by IVD biomechanical studies and strong citation bursts) proposed a series of mechanical criterions or benchmarks of native IVD tissue. Utilizing these benchmarks, subsequent repair strategies can be designed more effectively.
Methods of AF repair include suture, void filling, and biomimetic tissue engineering scaffolds.40 Void filling methods use injectable hydrogels or other materials to repair the defects in the AF. To reach better adhesion with native tissue, combining hydrogels with biocompatible cross-linkers is a feasible way, such as collagen cross-linked with riboflavin41 and fibrin cross-linked with genipin.42 Biomimetic tissue engineering could mimic the collagen fiber architecture of native AF using aligned fibrous scaffolds. The scaffolds can be produced by various techniques, such as electrospinning, collagen contraction, silk-fiber winding, among which electrospinning is preferred.43 A study by Nerurkar et al44 (in cluster #0) had raised considerable attentions with 17.5 citations per year and high betweenness centrality. They developed an aligned electrospun nanofibrous scaffolds seeded with mesenchymal stem cells for AF repair. This scaffold succeeded to generate angle-ply multi-lamellar tissues that replicate the organized structure of the AF, as well as the anisotropic mechanical properties. To uncover the role of oriented lamellar structure, their later work constructed three bilayers with three different fiber orientations to perform uniaxial tensile testing.45 The experimental data was then applied to the constitutive model. Results showed that interlamellar shearing maximizes the reinforcement of the tensile response when fibers in adjacent lamellae were aligned in opposing directions. This finding highlighted the role of organized lamellar structure in the success of AF repair.45 In addition, compared to scaffolds without organized lamellar structure, biomimetic scaffolds with lamellar structure may not require adhesion or void filling.46 Hence, biomimetic tissue engineering scaffolds may be a promising field of AF repair.
In addition to AF repair, there is a remarkable expansion in the studies about NP replacement as well. To date, many injectable natural and synthetic materials for NP replacement have been developed. Biocompatible natural materials such as alginate, agarose, hyaluronan, collagen, chitosan and cellulose can provide favorable environment for IVD cells.26 However, researches in recent years have found the common limitation of them, weakness in mechanics. Therefore, great efforts have been made to develop composite materials with improved mechanical properties, such as cellulose/chitosan,47 fibrin/Silk48 and gelatin/hyaluronic acid.49 In one study, an injectable bioinspired formulation of cellulose nanofibril-reinforced Chitosan had been proposed for NP replacement and exhibited an increase of the elastic modulus with the increase of the cellulose nanofibril content.47 This approach was also appropriate for AF repair.50 These findings highlighted the importance of composite materials. In addition, due to well controlled properties, a variety of synthetic polymeric materials have also been investigated as potential NP substitutes, including in situ hydrating polymers and in situ forming polymers.40 While biomaterial-based therapy has shown promise in preclinical studies, it has not yet been well demonstrated clinically, leaving a gap in IVD repair.
Over several decades, numerous outstanding papers in IVD biomechanics have been published. Combining validated metrics, the present study has found the most representative literatures (Table 2, Tables 4 and 5). These literatures contribute to several fields, including mechanical testing of normal and pathological IVDs, mechanical evaluation of new repair strategies and development of finite element model. Landmark studies on IVD repair have been discussed. Other landmark studies will be discussed in the following paragraphs.
Understanding the mechanical properties of normal and degenerative IVDs is key to IVD biomechanics. Many classic studies, the majority of which were found in cluster #1, contributed a lot to this area. As cornerstones of further studies, most of them had high annual average citation counts and betweenness centrality (Tables 2 and 6). Specifically, Wilke et al9 (with 37.63 overall citations per year) conducted an in vivo direct measurement of intradiscal pressure in a volunteer male orthopedist. The result showed that the resting intradiscal pressure is roughly 0.1–0.24 MPa at night and increases to 0.3–1.1 MPa when standing or sitting, and to 2.3 MPa when lifting a 20-kg weight in a flexed position. This study was considered a supplement to Nachemson’s earlier works.51 MacLean et al32 (in Cluster #1) performed a mechanobiological in vivo studies, which was been mentioned before for their highest betweenness centrality and crucial position in the co-citation network to link the cluster #1 and #0. The results showed that responses in the disc cell to mechanical loading was dependent on duration of loading and there were distinct responses between the AF and NP. Three studies with high betweenness centrality investigated the multi-scale biomechanical behaviors of IVDs.14,33,52 Elliott et al14 (in Cluster #1, 0.12 in centrality) provided evidences that compared to the inner AF, the outer AF was more effective to withstand the circumferential stresses, according to higher circumferential tensile modulus in the linear area. Holzapfel et al33 (in Cluster #1, 0.10 in centrality) demonstrated that at the shallow radial position (≤3.9±0.21 mm) of AF, collagen fiber angles depend predominately on the circumferential position. The fiber angle was smallest in the midsagittal ventral position (23°) and biggest in the midsagittal dorsal position (47°). This studies also provided valuable mechanical information about nonlinearity, anisotropy, heterogeneity and viscoelasticity at the scale of single lamellar. Guilak et al52 (in Cluster #1, 0.10 in centrality) characterized the micromechanical properties of IVD cells using alginate as the 3D matrix and provided available evidence that NP cells were more viscous and stiffer than AF cells, suggesting the existence of biomechanical distinction between cell types. A recent review article by Newell et al5 (in cluster #5), with highest citations contributed by IVD biomechanical studies and strongest citation bursts, amalgamated many experimental testing techniques adopted by biomechanical research to instruct experimentalists and computational modelers. It can be thought to be one of the most important landmark studies in IVD biomechanics.
Mechanical pathology of disc degeneration was investigated by numerous landmark studies as well. Specifically, Adam et al53 (17.37 overall citations per year) compared the distribution of stress between normal, aged and degenerated IVDs. The results showed that degeneration reduced the diameter of the functional NP by approximately 50{64d42ef84185fe650eef13e078a399812999bbd8b8ee84343ab535e62a252847}, and the pressure insides this region by 30{64d42ef84185fe650eef13e078a399812999bbd8b8ee84343ab535e62a252847}. Instead, both the width of the functional annulus and the height of compressive stress peaks within it increased. Another study with high betweenness centrality by Adam et al54 (in cluster #3, 0.10 in centrality) reached a similar conclusion that minor injury of CEPs may lead to a decrease in intradiscal pressures and stress concentrations in the AF. These findings supported the role of high ‘stress’ concentrations within the AF in the development of IVD degeneration. A large in vivo study conducted by Sato et al55 (15.54 overall citations per year) corroborated this conclusion. They demonstrated that degeneration does influence the intradiscal pressure and there exists a clear dose-effect relationship between degenerative grade and intradiscal pressure. In the field of mechanical pathology, a review article by Vergroesen et al,16 with 36.5 overall citations per year, 40 citations contributed by IVD biomechanical studies and strong citation bursts, provided a comprehensive discussion about the interaction between biomechanics, extracellular matrix, and cells during degeneration.
Adjacent-level effects had also been investigated by several landmark biomechanical studies with high annual average citation counts. Adjacent segment disease (ASD) is a long-term outcome of surgery. Early epidemiological report of ASD by Hilibrand et al56 had raised significant attentions. Naturally, a biomechanical study should be performed to uncover the pathogenesis. In 2002, Eck et al57 (with 23.38 overall citations per year) performed an in vitro study. With upper cervical spine segment stabilized, the intradiscal pressures during flexion increased by 73.2{64d42ef84185fe650eef13e078a399812999bbd8b8ee84343ab535e62a252847} at C4-C5, and by 45.3{64d42ef84185fe650eef13e078a399812999bbd8b8ee84343ab535e62a252847} at C6-C7. A strong link between impaired mechanical function and adjacent segment disease was established by these findings. Due to adjacent-level effects of spinal fusion, non-fusion motion preservation devices were developed. Charite was the first lumbar implant commercially available.58 Since then, more artificial discs were designed. Hence, comparison of mechanical properties between the substitute and native IVDs became a major consideration. In 2005, Goel et al59 (in cluster #4, with 35 citations and strong citation bursts contributed by IVD biomechanical studies) investigated the biomechanical effects of the Charité artificial disc across the implanted and adjacent segments by a hybrid test method. The result showed that the Charité increased the motion at the implanted level, and decreased the motions at the adjacent levels, which led to corresponding changes in loads. These findings conformed the biomechanical advantage of motion preservation devices. Compared to spinal fusion, short-term superiority of total disc replacement has also been proved by longitudinal follow-up clinical studies.60,61 However, there are still concerns about the long-term outcome, such as reoperations.62
In vivo and in vitro biomechanical studies investigating native IVDs, and biomaterials have yielded many successes. Meanwhile, finite element (FE) analysis, a research method based on computer, has also experienced rapid growth. Compared to in vitro or in vivo approaches, computational methods can offer cost-effective solutions with less ethical concerns. The earliest of them was completed by Belytschko et al63 in 1974 investigating stress analysis of IVDs. In 2006, Rohlmann et al29 (in cluster #0, with 43 citations as well as strong citation bursts contributed by IVD biomechanical studies) developed a three-dimensional nonlinear FE model to investigate the influence of disc degeneration on the mechanics of lumbar motion segment. In the same year, Schmidt et al64 (in cluster #0, with 40 citations as well as strong citation bursts contributed by IVD biomechanical studies) developed a method to calibrating the FE model of human lumbar AF. Since then, FE analysis had been adopted by numerous studies to investigate IVD biomechanics. When it came to 2014, Dreischarf et al28 (in cluster #2, with 44 citations as well as strong citation bursts contributed by IVD biomechanical studies) compared the relative predictive power of eight well-established FE models of L1–L5. The result showed that the median of all FE model predictions was always relatively close to the in vitro median values of the intervertebral rotations, intradiscal pressure and facet joint forces. Studies by Dreischarf et al,28 Rohlmann et al29 and Schmidt et al64 had become the references with the highest citation counts contributed by IVD biomechanical papers, except for review article written by Newell et al5 Accordingly, FE model studies may have made important contributions to our understanding of IVD biomechanics.
Considering the list of the 10 references with the highest citations contributed by IVD biomechanical studies (Table 4), half focused on developing models or new methods of biomechanical tests. These studies provided principles for IVD biomechanical experiments. For example, based on Newell et al’s review of disc composition and functional anatomy,5 Wang et al determined the thickness of cartilaginous endplates in their finite element model.65 In addition, Newell et al summarized characteristics of IVD during bending and axial rotation tests, highlighting the “neutral zone” where the torque is zero (hysteresis), and Bezci et al combined the “zero torque location” and “zero rotation location” to form the concept of a “hysteresis loop.”66 The shape change of the “hysteresis loop” was then used to explore the combined effect of axial compressive preload and rotation angle on the mechanical behavior of IVDs.
The major contributors in IVD biomechanics were very concentrated. Wilke HJ, Elliott DM, and Iatridis JC have been the most productive authors of papers of IVD biomechanics, and Adams MA, Panjabi MM, and Wilke HJ were the most cited authors of identified references (Table 7). Particularly, Adams MA ranked as the most cited author of included references in each 5-year slice. Spine, Eur Spine J, and J Biomech published the most papers of IVD biomechanics and were the journals cited most by original papers as well (Table 8, Figure 6). Among the top 10 references with the highest citation counts contributed by papers of IVD biomechanics, half were published in J Biomech. In the lists of references with the highest betweenness centrality and papers with the most annual average citation counts, respectively, half and 40{64d42ef84185fe650eef13e078a399812999bbd8b8ee84343ab535e62a252847} were published in Spine, respectively. Regardless of the timespan selected, the United States was the most productive country. However, publications from the People’s Republic of China have increased rapidly over time.
Conclusion
The major research topic of IVD biomechanics may have evolved from mechanobiology to mechanical repair strategies of IVDs, and the latter has been identified as an emerging trend in IVD biomechanics, which is a major finding of the present study.
Landmark studies identified have contributed to several fields, including mechanical testing of normal and pathological IVDs, mechanical evaluation of new repair strategies and development of FE model. In the field of mechanical physiology, in vivo study by Wilke et al,9 with the highest annual average citations, provided valuable data of intradiscal pressure by direct measurement. Review article for biomechanical testing techniques by Newell et al,5 had the most citations and strongest bursts of citations contributed by IVD biomechanics. In vivo study by MacLean et al32 (in Cluster #1), with highest betweenness centrality, uncovered the mechanobiological distinction between NP and AF cell, which should be considered as an important bridge in topics evolution. In the field of mechanical pathology, review article by Vergroesen et al16 highlighted the role of vicious circle between biomechanics, extracellular matrix, and cells in the development of IVD degeneration. In vitro study by Adam et al53 demonstrated the crucial role of ‘stress’ concentrations in IVD degeneration. In term of IVD repair, Nerurkar et al had reviewed the benchmarks of native IVDs39 and developed an aligned electrospun nanofibrous scaffolds for AF repair,44 both of which are representative studies. Finally, FE model studies by Dreischarf et al,28 Rohlmann et al29 and Schmidt et al,64 with high citation counts contributed by IVD biomechanical papers, should also be regarded as landmark studies. Additionally, the present study explored the major contributors to IVD biomechanics. Adams MA was the most cited author among the references providing the most literature basis for IVD biomechanical research. Spine, Eur Spine J, and J Biomech were the major journals for both original papers and references. The United States has made significant contributions to the field; however, Chinese researchers are catching up.
Acknowledgments
We thank Wangjing Hospital of China Academy of Chinese Medical Sciences for supporting this work. DZ, MF, and WL are co–first authors.
Funding
This work was supported by the Beijing Natural Science Foundation (no. 7214297) and the Innovation Team and Talents Cultivation Program of National Administration of Traditional Chinese Medicine (no. ZYYCXTD-C-202003).
Disclosure
The authors report no conflicts of interest in this work.
References
1. Safiri S, Kolahi AA, Hoy D, et al. Global, regional, and national burden of neck pain in the general population, 1990–2017: systematic analysis of the Global Burden of Disease Study 2017. BMJ. 2020;368:m791. doi:10.1136/bmj.m791
2. Chen S, Chen M, Wu X, et al. Global, regional and national burden of low back pain 1990–2019: a systematic analysis of the Global Burden of Disease study 2019. J Orthop Translat. 2022;32:49–58. doi:10.1016/j.jot.2021.07.005
3. Ravindra VM, Senglaub SS, Rattani A, et al. Degenerative lumbar spine disease: estimating global incidence and worldwide volume. Global Spine J. 2018;8(8):784–794. doi:10.1177/2192568218770769
4. Urban JP, Roberts S. Degeneration of the intervertebral disc. Arthritis Res Ther. 2003;5(3):120–130. doi:10.1186/ar629
5. Newell N, Little JP, Christou A, Adams MA, Adam CJ, Masouros SD. Biomechanics of the human intervertebral disc: a review of testing techniques and results. J Mech Behav Biomed Mater. 2017;69:420–434. doi:10.1016/j.jmbbm.2017.01.037
6. Whatley BR, Wen X. Intervertebral disc (IVD): structure, degeneration, repair and regeneration. Mater Sci Eng C. 2012;32(2):61–77. doi:10.1016/j.msec.2011.10.011
7. Wei Q, Zhang X, Zhou C, Ren Q, Zhang Y. Roles of large aggregating proteoglycans in human intervertebral disc degeneration. Connect Tissue Res. 2019;60(3):209–218. doi:10.1080/03008207.2018.1499731
8. Johannessen W, Elliott DM. Effects of degeneration on the biphasic material properties of human nucleus pulposus in confined compression. Spine. 2005;30(24):E724–9. doi:10.1097/01.brs.0000192236.92867.15
9. Wilke HJ, Neef P, Caimi M, Hoogland T, Claes LE. New in vivo measurements of pressures in the intervertebral disc in daily life. Spine. 1999;24(8):755–762. doi:10.1097/00007632-199904150-00005
10. Neidlinger-Wilke C, Galbusera F, Pratsinis H, et al. Mechanical loading of the intervertebral disc: from the macroscopic to the cellular level. Eur Spine J. 2014;23(Suppl 3):S333–43. doi:10.1007/s00586-013-2855-9
11. Marchand F, Ahmed AM. Investigation of the laminate structure of lumbar disc anulus fibrosus. Spine. 1990;15(5):402–410. doi:10.1097/00007632-199005000-00011
12. Choi Y, Park MH, Lee K. Tissue engineering strategies for intervertebral disc treatment using functional polymers. Polymers. 2019;11(5):872. doi:10.3390/polym11050872
13. Michalek AJ, Buckley MR, Bonassar LJ, Cohen I, Iatridis JC. Measurement of local strains in intervertebral disc anulus fibrosus tissue under dynamic shear: contributions of matrix fiber orientation and elastin content. J Biomech. 2009;42(14):2279–2285. doi:10.1016/j.jbiomech.2009.06.047
14. Elliott DM, Setton LA. Anisotropic and inhomogeneous tensile behavior of the human anulus fibrosus: experimental measurement and material model predictions. J Biomech Eng. 2001;123(3):256–263. doi:10.1115/1.1374202
15. Guerin HL, Elliott DM. Quantifying the contributions of structure to annulus fibrosus mechanical function using a nonlinear, anisotropic, hyperelastic model. J Orthop Res. 2007;25(4):508–516. doi:10.1002/jor.20324
16. Vergroesen PP, Kingma I, Emanuel KS, et al. Mechanics and biology in intervertebral disc degeneration: a vicious circle. Osteoarthritis Cartilage. 2015;23(7):1057–1070. doi:10.1016/j.joca.2015.03.028
17. Lu X, Zou F, Lu F, Ma X, Xia X, Jiang J. Bibliometric and visualized analysis of current research trends in the finite element analysis of lumbar spine. Interdiscip Neurosurg. 2021;26:101350. doi:10.1016/j.inat.2021.101350
18. Xie L, Chen Z, Wang H, Zheng C, Jiang J. Bibliometric and Visualized analysis of scientific publications on atlantoaxial spine surgery based on web of science and VOSviewer. World Neurosurg. 2020;137:435–442. doi:10.1016/j.wneu.2020.01.171
19. Yin M, Xu C, Ma J, Ye J, Mo W. A bibliometric analysis and visualization of current research trends in the treatment of cervical spondylotic myelopathy. Global Spine J. 2021;11(6):988–998. doi:10.1177/2192568220948832
20. Donnally CJ
21. Chen CM, CiteSpace II. Detecting and visualizing emerging trends and transient patterns in scientific literature. Article. J Am Soc Inf Sci Technol. 2006;57(3):359–377. doi:10.1002/asi.20317
22. Small H. Co-citation in the scientific literature: a new measure of the relationship between two documents. J Am Soc Inf Sci. 1973;24(4):265–269. doi:10.1002/asi.4630240406
23. Chen C, Hu Z, Liu S, Tseng H. Emerging trends in regenerative medicine: a scientometric analysis in CiteSpace. Expert Opin Biol Ther. 2012;12(5):593–608. doi:10.1517/14712598.2012.674507
24. Chen C, Ibekwe-SanJuan F, Hou J. The structure and dynamics of cocitation clusters: a multiple-perspective cocitation analysis. J Am Soc Inf Sci Technol. 2010;61(7):1386–1409. doi:10.1002/asi.21309
25. Brown T, Hansen RJ, Yorra AJ. Some mechanical tests on the lumbosacral spine with particular reference to the intervertebral discs; a preliminary report. J Bone Joint Surg Am. 1957;39–a(5):1135–1164. doi:10.2106/00004623-195739050-00014
26. Iatridis JC, Nicoll SB, Michalek AJ, Walter BA, Gupta MS. Role of biomechanics in intervertebral disc degeneration and regenerative therapies: what needs repairing in the disc and what are promising biomaterials for its repair? Spine J. 2013;13(3):243–262. doi:10.1016/j.spinee.2012.12.002
27. Setton LA, Chen J. Cell mechanics and mechanobiology in the intervertebral disc. Spine. 2004;29(23):2710–2723. doi:10.1097/01.brs.0000146050.57722.2a
28. Dreischarf M, Zander T, Shirazi-Adl A, et al. Comparison of eight published static finite element models of the intact lumbar spine: predictive power of models improves when combined together. J Biomech. 2014;47(8):1757–1766. doi:10.1016/j.jbiomech.2014.04.002
29. Rohlmann A, Zander T, Schmidt H, Wilke HJ, Bergmann G. Analysis of the influence of disc degeneration on the mechanical behaviour of a lumbar motion segment using the finite element method. J Biomech. 2006;39(13):2484–2490. doi:10.1016/j.jbiomech.2005.07.026
30. Galbusera F, Schmidt H, Noailly J, et al. Comparison of four methods to simulate swelling in poroelastic finite element models of intervertebral discs. J Mech Behav Biomed Mater. 2011;4(7):1234–1241. doi:10.1016/j.jmbbm.2011.04.008
31. O’Connell GD, Jacobs NT, Sen S, Vresilovic EJ, Elliott DM. Axial creep loading and unloaded recovery of the human intervertebral disc and the effect of degeneration. J Mech Behav Biomed Mater. 2011;4(7):933–942. doi:10.1016/j.jmbbm.2011.02.002
32. MacLean JJ, Lee CR, Alini M, Iatridis JC. The effects of short-term load duration on anabolic and catabolic gene expression in the rat tail intervertebral disc. J Orthop Res. 2005;23(5):1120–1127. doi:10.1016/j.orthres.2005.01.020
33. Holzapfel GA, Schulze-Bauer CA, Feigl G, Regitnig P. Single lamellar mechanics of the human lumbar anulus fibrosus. Biomech Model Mechanobiol. 2005;3(3):125–140. doi:10.1007/s10237-004-0053-8
34. Kim S, Uroz M, Bays JL, Chen CS. Harnessing mechanobiology for tissue engineering. Dev Cell. 2021;56(2):180–191. doi:10.1016/j.devcel.2020.12.017
35. Fujii K, Lai A, Korda N, et al. Ex-vivo biomechanics of repaired rat intervertebral discs using genipin crosslinked fibrin adhesive hydrogel. J Biomech. 2020;113:110100. doi:10.1016/j.jbiomech.2020.110100
36. Panebianco CJ, Meyers JH, Gansau J, Hom WW, Iatridis JC. Balancing biological and biomechanical performance in intervertebral disc repair: a systematic review of injectable cell delivery biomaterials. Eur Cell Mater. 2020;40:239–258. doi:10.22203/eCM.v040a15
37. Maidhof R, Alipui DO, Rafiuddin A, Levine M, Grande DA, Chahine NO. Emerging trends in biological therapy for intervertebral disc degeneration. Discov Med. 2012;14(79):401–411.
38. Moriguchi Y, Alimi M, Khair T, et al. Biological treatment approaches for degenerative disk disease: a literature review of in vivo animal and clinical data. Global Spine J. 2016;6(5):497–518. doi:10.1055/s-0036-1571955
39. Nerurkar NL, Elliott DM, Mauck RL. Mechanical design criteria for intervertebral disc tissue engineering. J Biomech. 2010;43(6):1017–1030. doi:10.1016/j.jbiomech.2009.12.001
40. Bowles RD, Setton LA. Biomaterials for intervertebral disc regeneration and repair. Biomaterials. 2017;129:54–67. doi:10.1016/j.biomaterials.2017.03.013
41. Grunert P, Borde BH, Towne SB, et al. Riboflavin crosslinked high-density collagen gel for the repair of annular defects in intervertebral discs: an in vivo study. Acta Biomater. 2015;26:215–224. doi:10.1016/j.actbio.2015.06.006
42. Schek RM, Michalek AJ, Iatridis JC. Genipin-crosslinked fibrin hydrogels as a potential adhesive to augment intervertebral disc annulus repair. Eur Cell Mater. 2011;21:373–383. doi:10.22203/ecm.v021a28
43. Chu G, Shi C, Wang H, Zhang W, Yang H, Li B. Strategies for annulus fibrosus regeneration: from biological therapies to tissue engineering. Front Bioeng Biotechnol. 2018;6:90. doi:10.3389/fbioe.2018.00090
44. Nerurkar NL, Baker BM, Sen S, Wible EE, Elliott DM, Mauck RL. Nanofibrous biologic laminates replicate the form and function of the annulus fibrosus. Nat Mater. 2009;8(12):986–992. doi:10.1038/nmat2558
45. Nerurkar NL, Mauck RL, Elliott DM. Modeling interlamellar interactions in angle-ply biologic laminates for annulus fibrosus tissue engineering. Biomech Model Mechanobiol. 2011;10(6):973–984. doi:10.1007/s10237-011-0288-0
46. Buckley CT, Hoyland JA, Fujii K, Pandit A, Iatridis JC, Grad S. Critical aspects and challenges for intervertebral disc repair and regeneration-Harnessing advances in tissue engineering. JOR Spine. 2018;1(3):e1029. doi:10.1002/jsp2.1029
47. Doench I, Torres-Ramos MEW, Montembault A, et al. Injectable and gellable chitosan formulations filled with cellulose nanofibers for intervertebral disc tissue engineering. Polymers. 2018;10(11):1202. doi:10.3390/polym10111202
48. Frauchiger DA, May RD, Bakirci E, et al. Genipin-enhanced fibrin hydrogel and novel silk for intervertebral disc repair in a loaded bovine organ culture model. J Funct Biomater. 2018;9(3):40. doi:10.3390/jfb9030040
49. Chen P, Ning L, Qiu P, et al. Photo-crosslinked gelatin-hyaluronic acid methacrylate hydrogel-committed nucleus pulposus-like differentiation of adipose stromal cells for intervertebral disc repair. J Tissue Eng Regen Med. 2019;13(4):682–693. doi:10.1002/term.2841
50. Doench I, Ahn Tran T, David L, et al. Cellulose nanofiber-reinforced chitosan hydrogel composites for intervertebral disc tissue repair. Biomimetics. 2019;4(1):19. doi:10.3390/biomimetics4010019
51. Nachemson A, Morris JM. In vivo measurements of intradiscal pressure. discometry, a method for the determination of pressure in the lower lumbar discs. J Bone Joint Surg Am. 1964;46:1077–1092. doi:10.2106/00004623-196446050-00012
52. Guilak F, Ting-Beall HP, Baer AE, Trickey WR, Erickson GR, Setton LA. Viscoelastic properties of intervertebral disc cells. Identification of two biomechanically distinct cell populations. Spine. 1999;24(23):2475–2483. doi:10.1097/00007632-199912010-00009
53. Adams MA, McNally DS, Dolan P. ‘Stress’ distributions inside intervertebral discs. The effects of age and degeneration. J Bone Joint Surg Br. 1996;78(6):965–972. doi:10.1302/0301-620x78b6.1287
54. Adams MA, Freeman BJ, Morrison HP, Nelson IW, Dolan P. Mechanical initiation of intervertebral disc degeneration. Spine. 2000;25(13):1625–1636. doi:10.1097/00007632-200007010-00005
55. Sato K, Kikuchi S, Yonezawa T. In vivo intradiscal pressure measurement in healthy individuals and in patients with ongoing back problems. Spine. 1999;24(23):2468–2474. doi:10.1097/00007632-199912010-00008
56. Hilibrand AS, Carlson GD, Palumbo MA, Jones PK, Bohlman HH. Radiculopathy and myelopathy at segments adjacent to the site of a previous anterior cervical arthrodesis. J Bone Joint Surg Am. 1999;81(4):519–528. doi:10.2106/00004623-199904000-00009
57. Eck JC, Humphreys SC, Lim TH, et al. Biomechanical study on the effect of cervical spine fusion on adjacent-level intradiscal pressure and segmental motion. Spine. 2002;27(22):2431–2434. doi:10.1097/00007632-200211150-00003
58. Othman YA, Verma R, Qureshi SA. Artificial disc replacement in spine surgery. Ann Transl Med. 2019;7(Suppl 5):S170. doi:10.21037/atm.2019.08.26
59. Goel VK, Grauer JN, Patel T, et al. Effects of charité artificial disc on the implanted and adjacent spinal segments mechanics using a hybrid testing protocol. Spine. 2005;30(24):2755–2764. doi:10.1097/01.brs.0000195897.17277.67
60. Zou S, Gao J, Xu B, Lu X, Han Y, Meng H. Anterior cervical discectomy and fusion (ACDF) versus cervical disc arthroplasty (CDA) for two contiguous levels cervical disc degenerative disease: a meta-analysis of randomized controlled trials. Eur Spine J. 2017;26(4):985–997. doi:10.1007/s00586-016-4655-5
61. Zigler J, Gornet MF, Ferko N, Cameron C, Schranck FW, Patel L. Comparison of lumbar total disc replacement with surgical spinal fusion for the treatment of single-level degenerative disc disease: a meta-analysis of 5-year outcomes from randomized controlled trials. Global Spine J. 2018;8(4):413–423. doi:10.1177/2192568217737317
62. Laugesen LA, Paulsen RT, Carreon L, Ernst C, Andersen M. Patient-reported outcomes and revision rates at a mean follow-up of 10 years after lumbar total disc replacement. Spine. 2017;42(21):1657–1663. doi:10.1097/brs.0000000000002174
63. Belytschko T, Kulak RF, Schultz AB, Galante JO. Finite element stress analysis of an intervertebral disc. J Biomech. 1974;7(3):277–285. doi:10.1016/0021-9290(74)90019-0
64. Schmidt H, Heuer F, Simon U, et al. Application of a new calibration method for a three-dimensional finite element model of a human lumbar annulus fibrosus. Clin Biomech. 2006;21(4):337–344. doi:10.1016/j.clinbiomech.2005.12.001
65. Wang K, Jiang DC, Wang DL, Wang H, Niu DW. The biomechanical influence of anterior vertebral body osteophytes on the lumbar spine: a finite element study. Spine J. 2018;18(12):2288–2296. doi:10.1016/j.spinee.2018.07.001
66. Bezci SE, Klineberg EO, O’Connell GD. Effects of axial compression and rotation angle on torsional mechanical properties of bovine caudal discs. J Mech Behav Biomed Mater. 2018;77:353–359. doi:10.1016/j.jmbbm.2017.09.022
67. Norman R, Wells R, Neumann P, Frank J, Shannon H, Kerr M. A comparison of peak vs cumulative physical work exposure risk factors for the reporting of low back pain in the automotive industry. Clin Biomech. 1998;13(8):561–573. doi:10.1016/s0268-0033(98)00020-5
68. Casaroli G, Villa T, Bassani T, Berger-Roscher N, Wilke HJ, Galbusera F. Numerical prediction of the mechanical failure of the intervertebral disc under complex loading conditions. Materials. 2017;10(1):31. doi:10.3390/ma10010031
69. McNally DS. The objectives for the mechanical evaluation of spinal instrumentation have changed. Eur Spine J. 2002;11(Suppl 2):S179–85. doi:10.1007/s00586-002-0435-5
70. Noailly J, Ambrosio L, Elizabeth Tanner K, Planell JA, Lacroix D. In silico evaluation of a new composite disc substitute with a L3–L5 lumbar spine finite element model. Eur Spine J. 2012;21(Suppl 5):S675–87. doi:10.1007/s00586-011-1716-7
71. Tamoud A, Zaïri F, Mesbah A, Zaïri F. A multiscale and multiaxial model for anisotropic damage and failure of human annulus fibrosus. Int J Mech Sci. 2021;205:106558. doi:10.1016/j.ijmecsci.2021.106558
72. Li Y, Fogel GR, Liao Z, Tyagi R, Zhang G, Liu W. Biomechanical analysis of two-level cervical disc replacement with a stand-alone U-shaped disc implant. Spine. 2017;42(20):E1173–e1181. doi:10.1097/brs.0000000000002128
73. Pritchard S, Erickson GR, Guilak F. Hyperosmotically induced volume change and calcium signaling in intervertebral disk cells: the role of the actin cytoskeleton. Biophys J. 2002;83(5):2502–2510. doi:10.1016/s0006-3495(02)75261-2
74. Huang RC, Wright TM, Panjabi MM, Lipman JD. Biomechanics of nonfusion implants. Orthop Clin North Am. 2005;36(3):271–280. doi:10.1016/j.ocl.2005.02.010
75. Schmidt H, Shirazi-Adl A, Galbusera F, Wilke HJ. Response analysis of the lumbar spine during regular daily activities—a finite element analysis. J Biomech. 2010;43(10):1849–1856. doi:10.1016/j.jbiomech.2010.03.035
76. Guerin HA, Elliott DM. Degeneration affects the fiber reorientation of human annulus fibrosus under tensile load. J Biomech. 2006;39(8):1410–1418. doi:10.1016/j.jbiomech.2005.04.007
77. Hsieh AH, Hwang D, Ryan DA, Freeman AK, Kim H. Degenerative anular changes induced by puncture are associated with insufficiency of disc biomechanical function. Spine. 2009;34(10):998–1005. doi:10.1097/BRS.0b013e31819c09c4
78. McNally DS, Shackleford IM, Goodship AE, Mulholland RC. In vivo stress measurement can predict pain on discography. Spine. 1996;21(22):2580–2587. doi:10.1097/00007632-199611150-00007
79. Olmarker K, Blomquist J, Strömberg J, Nannmark U, Thomsen P, Rydevik B. Inflammatogenic properties of nucleus pulposus. Spine. 1995;20(6):665–669. doi:10.1097/00007632-199503150-00006